Mycotoxins in animal production: Risks, prevention and solutions
Orffa, October 2023
General information mycotoxins
Mycotoxins are secondary metabolites that are produced by certain filamentous fungi. They are found to be common contaminants of food and feed sources. There are 3 main genera of fungi associated with the production of mycotoxins, which are Aspergillus, Fusarium and Penicillium. In general, these genera of fungi are not specifically harmful to plants, but under certain conditions (e.g. if they can enter the plant, favourable climate), mycotoxins can be produced during the growing season (CAST, 2003).
Mycotoxins can be produced at different stages of the production processing of plant material; before or after harvest, and during storage. Mycotoxins in feed can have negative effects on the animal, but can also pose a threat to human health when they are converted in animal products such as milk, eggs or meat (Gruber-Dorninger et al. 2019).

The contamination of crops with mycotoxins depends on different factors, such as environmental factors (e.g. temperature, humidity), but also plant-specific factors, as some crops are more susceptible to certain fungi. An important environmental factor is climate change: it is expected to increase the mycotoxin challenge, and extend it to regions that are currently less affected by mycotoxins (Russel et al. 2010). Examples of plant-specific factors that influence production of mycotoxins are bioavailability of micronutrients and insect damage (Milani, J.M. 2013).
In 2019, it was shown in a survey that 88% of all tested feed samples contained at least one mycotoxin (Gruber-Dorninger et al. 2019). Therefore, the economic impact of mycotoxins is severe. Economic effects can be related to animal mortality, disease, reduced production, but also crop losses and mycotoxin analysis (Zain, M.E. 2011). It is estimated that the USA spends approximately $418 million - $1.66 billion dollars yearly due to mycotoxin contamination of crops. This accounts for a mean cost of approximately $932 million dollars. Besides, efforts to reduce the mycotoxin challenge in the USA account for another $466 million dollars, and livestock losses account for around $6 million dollars. These costs do not yet include human health effects and highlight the severity of the mycotoxin problem (CAST, 2003).
The mycotoxins that are most relevant for animal production are aflatoxins, fumonisins, zearalenone, trichothecenes (deoxynivalenol, T-2 toxin) and ochratoxin (Richard, J.L. 2007).

Aflatoxins
Aflatoxins are produced by Aspergillus fungi, of which Aspergillus flavus is the main producer and, to a lesser extent, another producer can be A. parasiticus. There are multiple types of aflatoxins, but the most commonly detected in feed are the B1, B2, G1 and G2 (Atherstone et al. 2016).
In order to prevent the production of aflatoxins in the feed, it is important to store the materials at low temperature and low humidity. In general, temperatures of over 20°C or humidity of more than 14% increase the risk of aflatoxin contamination (Ominski et al. 1994). Besides, the feed should be kept at a cool, dry place without the presence of any insects, since insects can cause transmission of fungi (Richard, J.L. 2007). Also stresses to the crops before harvest are important to avoid, since such stressors (e.g. drought, heavy rains, inadequate drying before storage) can make the crop more susceptible to aflatoxin production (Lizárraga-Paulin et al. 2011).
Upon ingestion of aflatoxins, they will be absorbed in the gastrointestinal tract, enter the bloodstream and end up in the liver, where they will be metabolized. Aflatoxin B1 or B2 will be converted into the metabolites M1 and M2, which can be excreted or can end up in animal products such as milk (Atherstone et al. 2016). Aflatoxins are considered to have mutagenic, tumorigenic, carcinogenic and immunosuppressive effects (Benkerroum, N. 2020). In general, there are two categories of aflatoxin infections; acute or chronic aflatoxicosis (Lizárraga-Paulin et al. 2011). Acute aflatoxicosis occurs when high levels of aflatoxins are ingested in a short period (Yagudayev and Ray, 2023) and is associated with liver damage, vomiting, lethargy, weakness and anorexia (Domijan and Peraica, 2010). On the other hand, chronic aflatoxicosis occurs when exposure at low levels occurs over a longer period of time. This reduces animal productivity, disease resistance and the quality of animal products, and can induce damage to organs (liver) (Benkerroum, N. 2020).
Fumonisins
Fumonisins are produced by Fusarium fungi, mostly by the species Fusarium verticillioides and Fusarium proliferatum (Voss et al. 2007). Fumonisin B1 (FB1) and fumonisin B2 (FB2) are the most abundant types of this mycotoxin, but fumonisin B3 (FB3) is also recognized as member of this group of mycotoxins (Lino et al. 2006). In general, corn is a raw material that is often contaminated with fumonisins, while sorghum and rice show contamination on occasion (CAST, 2003). Some factors that appear to stimulate production of fumonisins are heat, moisture, stress and damage to the plants caused by insects, while the plant is still growing. When high levels of fumonisins are present, some kernels will show contamination by being whiter (called pink kernel rot) while others do not. Fumonisin concentrations seem to be very sensitive to storage conditions, so in order to prevent the production of fumonisins in stored feed, it’s important to screen the product before storage and keep it dry during storage, with moisture levels below 14% (Richard, J.L. 2007).
Fumonisins closely resemble the molecular structure of sphingosine, which is the backbone of cellular sphingolipids. Fumonisins have been shown to inhibit the ceramide synthase and sphingolipid metabolism, which causes an increase in levels of sphinganine (SA), which is the substrate of the enzyme’s sphingoid base and also of sphingosine (So). This is the general toxicity mechanism of fumonisins, it can cause damage to membrane structures which harms the animal (Merril et al. 2001). Toxicity of fumonisins is generally classified under oxidative stress, reproductive toxicity, autophagy, apoptosis and carcinogenic activity (Qu et al. 2022).
Zearalenone
Zearalenone is produced by Fusarium fungi, in particular by Fusarium graminearum, Fusarium oxysporum, Fusarium equisetum and Fusarium nivalis (Han et al. 2022).
In general, corn is an ingredient that is often contaminated by zearalenone, but some others such as barley, wheat, rye and sorghum can be affected by this mycotoxin as well (CAST, 2003). Zearalenone contamination in grains can sometimes be recognized, though not in all cases, by a pinkish colour, caused by the formation of a pink pigment together with contamination by zearalenone. Contamination occurs when there is high humidity and cool temperatures both before and after harvest. Since production of this mycotoxin occurs at cool temperatures, a cool storage location cannot protect the ingredients against zearalenone production (Richard, J.L. 2007).
In the animal, zearalenone is rapidly absorbed, after which hydroxylation takes place, resulting in the formation of α-zearalenone and β-zearalenone. Zearalenone, but also the reduced form of zearalenone, called zearalenol, is known to have estrogenic activity, causing it to be the main causative agent of reproductive disorders. Zearalenone has been shown to be genotoxic, hepatotoxic and haematotoxic. It interferes with the endocrine system, negatively affects immunity and can cause reproductive and developmental toxicity (Zinedine et al. 2007).

Trichothecenes
The trichothecenes form a family of more than 200 different types of mycotoxins. In general, this family can be subdivided into four groups: A, B, C or D, depending on their molecular structure. They all have a tricyclic 12,13-epoxytrichothec-9-ene structure but differ in their side groups (McCormick, 2011). Within the family of trichothecenes, deoxynivalenol (DON) and T-2 toxin are among the most studied, due to their high occurrence and level of toxic effects (Li et al. 2022).
T-2 toxin is part of the type A trichothecenes, and is produced by Fusarium fungi, such as Fusarium soprotrichioides, Fusarium acuinatum and Fusarium poae. T-2 is a common contaminant of cereal grains, especially of corn, wheat and oats (Li et al. 2011). Production of T-2 toxins is favoured by cool temperatures or high humidity. Storage of ingredients at temperatures around 20°C increases T-2 toxin production, while warmer storage temperatures generally show lower contamination with this mycotoxin (Mateo et al. 2001). An indication of contamination could be white, or pink to red mould growth on the ingredients. Regarding storage, it is important to reduce humidity and presence of insects (Richard, J.L. 2007). T-2 is known for its high (acute) toxicity, as it can lead to diarrhoea, vomiting, lethargy, haemorrhages, weight loss, reduced immunity, necrosis, apoptosis, damage to cartilage and even death (Li et al. 2011).
Deoxynivalenol (DON) is produced by Fusarium fungi, of which the main producer is Fusarium graminearum (Yuen and Schoneweis, 2007). DON is part of the family of type B trichothecenes (Pestka, J.J. 2007). This mycotoxin is known to mostly affect corn but also other grains such as oats, barley and wheat (CAST, 2003). Optimal conditions for Fusarium graminearum and the associated production of DON are cool temperatures and a moist environment. Crop diseases that are associated with DON are ear rot and head blight, as in some cases, DON production seems to be essential for the Fusarium graminearum to cause these diseases. Ear rot can be recognized by a blanched look of the kernels or pink stains (pink scab). It is recommended to store the raw materials at low moisture levels, below 14%, and minimize presence of insects. When stored correctly, DON will not further accumulate (Richard, J.L. 2007).
DON is also called the vomitotoxin, referring to the fact that it can cause animals/humans to vomit upon ingestion. Exposure to DON can reduce growth performance (e.g. feed intake, weight gain) and it has been shown to exert cytotoxic, genotoxic, and carcinogenic effects. Also, DON reduces immune functioning in animals, and extremely high levels of DON can cause shock-like death (Sobrova et al. 2010).
Ochratoxin
Ochratoxin A is the most occurring member of the ochratoxin group, and it is produced by Aspergillus and Penicillium fungi. Some metabolites linked to ochratoxin A are ochratoxin B, ochratoxin C, ochratoxin α, and ochratoxin β. The highest producers of this mycotoxin are Aspergillus ochraceus and Penicillium verrucosum (CAST, 2003). Ochratoxin A is found on a wide range of crops, such as corn, rice and millet (Khoury and Atoui, 2010). It can be produced in some crops in the field, but the main production is found to occur during storage. Mould contamination in the crop can be recognized by yellow (when infected with Aspergillus ochraceus) or blue (when infected with Penicillium) colours, or sometimes even black colours. It can also occur that the mould is not detected by an unusual colour, but by an unusual smell. High moisture and high temperatures favour the production of ochratoxin A, therefore it is important to keep the ingredients dry and cool during storage (Richard, J.L. 2007).
Exposure to ochratoxin A is associated with hepatoxicity, nephrotoxicity, teratogenicity and immune suppression. It is known to cause oxidative stress and therefore oxidative stress-associated damage to, for example, DNA and lipids (Tao et al. 2018).

Emerging mycotoxins
Emerging mycotoxins can be defined as mycotoxins that are not routinely determined, nor regulated by legislation. Even though little attention is given to this group of mycotoxins, their incidence is increasing (Vaclavikova et al. 2013). Based on this definition, it can be expected that many mycotoxins with (potential) toxicity are part of this group. Some of the most studied emerging mycotoxins appear to be enniatins, beauvericin, fusaproliferin, culmorin, moniliformin, fusaric acid, emodin, alternariol, butanolide, tenuazonic acid, sterigmatocystin, mycophenolic acid and alternariol monomethyl ether. For many of the members of the emerging mycotoxin group, there are knowledge gaps, highlighting the need for more research and attention towards these mycotoxins, so that a risk assessment can be done (Gruber-Dorninger, 2017). When risk assessments of these mycotoxins have been made, it will be possible to set legislation and guidance levels around these mycotoxins (Kovalsky et al. 2016). The knowledge gaps are currently still present in all three main factors for risk assessment; occurrence, toxicity and toxicokinetics. For some of the emerging mycotoxins, such as enniatins, alternariol or moniliformin, toxicity data is available. However, this data mostly originates from in vitro studies, while in vivo data remains limited (Fraeyman et al. 2017).
Co-occurrence
It is possible that a feed sample is contaminated with more than one mycotoxin at a time, this is called a co-occurence. There can be different reasons for co-occurrence; several fungi are capable of producing more than one mycotoxin at the same time, feed (ingredients) can be contaminated with more than one fungi at a time, and final feed usually consists out of several ingredients (which can all contain different mycotoxins) (Smith et al. 2016). A recent survey showed that about 48% of all feed samples (7049 in total) contained two or more mycotoxins (Rodrigues and Nachrer, 2012). Most studies show synergistic or additive effects when there is co-occurrence of multiple mycotoxins, and these effects are generally regarding decreased animal performance. (Grenier and Oswald, 2011).
In general, it is considered difficult to interpret toxicity data of co-occurring mycotoxins. It is expected that mycotoxins, that have similar toxicity mechanisms, cause synergistic and additive effects (Speijers and Speijers, 2004). However, besides the toxicity of each mycotoxin separately, there are many factors that could influence the final toxicity of the co-occurring mycotoxins such as toxicokinetics, toxicodynamics, mechanism and chemistry in cells, and experimental design (Lee and Ryu, 2017).
It was shown, based on data from literature including 127 feed samples, that the most occurring combinations of mycotoxins are co-occurrence of aflatoxins and ochratoxin A (21%), aflatoxins and fumonisins (20%) and DON and zearalenone (13%). Other combinations of mycotoxins were also shown to co-occur, but are less frequent (Smith et al. 2016).

• Aflatoxins and ochratoxin A
The combination of aflatoxins and ochratoxin A was shown to cause additive effects in reducing the viability of kidney cells, increasing the levels of fragmented DNA, causing DNA damage and accordingly enhancing the expression of p53 and bcl-2 (Golli-Bennour et al. 2010). Another study however, showed that when combining aflatoxins and ochratoxin A, it could reduce the DNA damage in the liver and that ochratoxin A could reduce the acute aflatoxin-caused hepatotoxicity (Corcuera et al. 2015). Abdel-Wahhab (2015) showed that this combination caused synergistic effects on toxicity, by causing changes in the liver and kidney tissues.
• Aflatoxins and fumonisins
Toxicity of the co-occurrence of aflatoxins and fumonisins is not uniform across studies. Some studies, such as Dilkin et al. (2003), do not show an interaction between those two mycotoxins, while others, such as Orsi et al. (2007), show a synergy between the mycotoxins regarding liver and kidney injuries. They show effects regarding liver function, kidney weight, creatine and urea levels and the Sa/So ration (Orsi et al. 2007).
• DON and zearalenone
Since zearalenone has a distinct toxicity mechanism, via its estrogenic effects, toxicity of co-occurrence with this zearalenone is harder to interpret (Lee and Ryu, 2017). Bensassi et al. (2014) showed that the combination of zearalenone and DON causes antagonism in reducing viability of cells, by enhancing the mitochondrial apoptosis. The combination however, seemed to reduce the individual toxic effects of the mycotoxins (Bensassi et al. 2014). Another study showed an additive myelotoxic effect, meaning a suppression of bone marrow (Ficheux et al. 2012).

Mycotoxins in swine
Swine are considered to be very sensitive to mycotoxins (Zain et al. 2011). Feed for swine is usually composed of a high degree of cereal grains, which are a major source of aflatoxins. That, in combination with the fact that monogastric animals are considered to have the highest sensitivity for aflatoxins, causes aflatoxins to present a big challenge in swine diets. Especially the liver is a major target, causing liver necrosis, haemorrhages or hepatitis. Moreover, immunosuppression and other immune modulations caused by aflatoxins have been reported in swine, but also damage to kidneys, heart, lymph nodes, spleen and uterus (Popescu et al. 2022).
Swine are considered to have high sensitivity to fumonisins as well, with some of the main effects including pulmonary oedema, damage to the lungs, heart and liver (Freitas et al. 2012).
Swine are especially sensitive to the oestrogenic effects of zearalenone (Zain et al. 2011). Symptoms of zearalenone in swine are known to be severe and include vulvovaginitis, reduced progesterone levels, reduced fertility, smaller litters, swollen vulva (especially in piglets), and problems with oestrus (Zinedine et al. 2007).
Also for ochratoxins, swine are considered among the most sensitive species, with nephropathy as the main clinical symptom, and the associated decrease in production performance (body weight gain (BWG), FCR, mortality) (Freitas et al. 2012).
Although all animals are considered to be susceptible to DON, pigs are the most sensitive for this type of mycotoxin, with a rapid absorption of DON. Symptoms such as vomiting, feed refusal and decreased growth performance (BWG, FCR) are observed (Pestka, J.J. 2007).
T-2 toxin in swine is mainly related to symptoms like dermatitis, abortions, haemorrhages or nervous disorders (Freitas et al. 2012).

Mycotoxins in poultry
Poultry are very sensitive to aflatoxins, which can be explained by their liver metabolism transforming aflatoxins into toxic metabolites. Aflatoxins in poultry cause a decrease in growth performance (feed intake, BWG, FCR). In laying hens, dietary aflatoxins can be linked to reduced egg production and egg quality, and increased mortality. In general, some other symptoms of aflatoxins in poultry can be reduced immune response, lower development of the thymus and a small bursa of Fabricius (Chen et al. 2013).
Since poultry diets (especially in the USA) are often based on corn, which is likely to be contaminated with fumonisins, this type of mycotoxin is often present in poultry feed. However, poultry can be considered less sensitive to fumonisins, with main symptoms related to reduced weight gain, higher liver weight (indicating an increased need for detoxification), elevated ratio of sphinganine/sphingosine ratio (Sa/So), liver necrosis, hyperplasia and thymic cortical atrophy (Voss et al. 2007).
Compared to other species, poultry can be considered to have lower sensitivity for zearalenone. This could possibly be explained by the fact that natural oestrogen levels are higher in poultry, and these natural oestrogen possesses a higher affinity for binding to oestrogen receptors compared to zearalenone. However, poultry can still negatively be affected by dietary zearalenone, with common symptoms related to problems in the development of the testes/oviduct (Liu and Applegate, 2020), but also reduced growth performance, achondroplasia, reduced liver health or oxidative stress. Important to consider is that zearalenone metabolites can easily end up in animal products such as eggs (Wu et al. 2021).
Severity of toxicity related to dietary ochratoxin is dependent on the level of this mycotoxin in the feed. Ochratoxins in poultry are related to reduced bird performance (BWG, FCR, egg production in layers), lower quality eggs and feathers, increased mortality, nephrotoxicity, immune suppression and increased organ weight for liver, spleen and gizzard (Mehtab et al. 2021).
DON related losses in poultry production are significant. Symptoms related to DON in poultry are reduced growth performance, increased disease susceptibility (immune suppression), vomiting, skin irritations, nausea, reduced intestinal health and organ lesions (Awad et al. 2012).
Another frequently occurring mycotoxin in poultry feed is T-2, which can result in reduced weight gain, necrosis or oral inflammation (Patil et al. 2014).

Mycotoxins in ruminants
In general, it can be stated that ruminants are less sensitive to (some) mycotoxins than monogastric animals, due to their ability to degrade mycotoxins, to some extend, in the rumen. Degradation in the rumen can prevent the mycotoxin from being absorbed and entering the bloodstream (Dicostanzo et al. 1995).
Ruminants can still be negatively affected by dietary aflatoxins, especially by AFB1. After ingestion of high amounts of this mycotoxin, it will appear in the milk in the form of AFM1. Symptoms related to aflatoxins in ruminants are feed refusal, reduced growth performance (BWG, FCR), anaemia, haemorrhages, abortions, immune suppression, rectal prolapse and listlessness (Cassel et al. 2001).
Degradation of fumonisins by ruminal microorganisms has been shown to be limited, even though ruminants are considered less susceptible for this mycotoxin. Some symptoms of fumonisins in ruminants can include toxicity for liver and kidneys, alteration in levels of serum enzymes (such as cholesterol, aspartate aminotransferase and lactate dehydrogenase) and an increased Sa/So ratio (EFSA Contam Panel, 2018).
Ruminants are not as susceptible to zearalenone as pigs, but similarly to poultry, ruminants can still be negatively affected by this mycotoxin. This is usually related to problems with the reproduction and genital organs, alteration in hormone levels (progesterone and luteinising hormone) and reduced milk yield (Yiannikouris and Jouany, 2002).
Toxicity of ochratoxins in ruminants is low, since the ruminal microorganisms can convert ochratoxin A into less-toxic metabolites (OTA α) (Yiannikouris and Jouany, 2002). Severe contamination by ochratoxin can still affect ruminants, with symptoms such as reduced feed intake, anorexia, diarrhoea and reduced milk yield in lactating animals (Mobashar et al. 2010).
Toxicity of DON in ruminants is lower compared to toxicity in swine and poultry (Pestka, J.J. 2007). Both DON and T-2 toxin can be partly degraded by the ruminal microorganisms. General symptoms of trichothecenes in ruminants, however, include feed refusal, reduced body weight, immune suppression, tissue necrosis and haemorrhages (Mostrom and Jacobsen, 2020).

Mycotoxins in aquaculture
The aquacultural industry has been using increased levels of plant-based ingredients, to replace fish meal. This contributes to an increased risk of mycotoxin contaminated feed, posing a significant challenge to the aquaculture industry. In general, it can be stated that the toxic dosages and effects of mycotoxins differ between the different aquacultural species (Matejova et al. 2017).
Aflatoxin was one of the first types of mycotoxins studied within the aquacultural sector. Similar to the toxicity effects in land-animals, aflatoxins are associated with carcinogenic properties in fish. Besides, immune suppression is a common symptom of aflatoxicosis in fish, causing the fish to be more prone for disease challenges (Spring and Burel, 2008).
In general, it can be stated that fish with lower body weights have a higher sensitivity to fumonisins compared to fish with higher body weights. Some common symptoms of fumonisins in fish diets include increased Sa/So ratio, lower body weights, increased susceptibility to diseases and higher mortality (Manning and Abbas, 2012).
Zearalenone toxicity in fish is based on only few studies. Overall, it can be stated, similar to other animals, that zearalenone in fish can exert oestrogenic effects by binding to oestrogen receptors (Pietsch et al. 2013).
In fish, dietary ochratoxin has been shown to mostly exert toxic effects on the liver and kidney (Matejova et al. 2017).
Symptoms of DON contaminated fish-diets include reduced growth performance (feed intake, BWG, FCR, Manning and Abbas, 2012), reduced immune response and increased oxidative stress (Anater et al. 2016). However, sensitivity to DON differs between species, with for example rainbow trout being very sensitive and channel catfish showing lower sensitivity (Manning and Abbas, 2012).
Literature on the toxic effects of T-2 toxin in fish is still limited, but it is suggested that this mycotoxin impairs growth performance (BWG, FCR, feed intake), causes haemorrhages of the intestine, increases mortality and alters the levels of haemoglobin and haematocrit (Matejova et al. 2017).

Important factors in prevention
As already discussed, many factors can affect the production of mycotoxins. Some of the most important effects originate from biological factors, environmental factors, harvesting, storage and distribution or processing (Bryden W.L. 2012).
Pre-harvest
Biological factors include susceptibility of the crop, spore load and compatibility of the mycotoxin producing fungi (Brydan W.L. 2012). In general, the biological factors can be classified under factors based on the interaction between the crop and the invading fungi (Tola and Kebede, 2016). Some plants are known to be more resistant to the production of high levels of mycotoxins. Such crops possess certain genes that are involved in inhibiting mycotoxin synthesis. Genetic modification allows researchers to develop crops that are less susceptible for fungal invasion and mycotoxin production. The development of such crops could contribute to reducing the mycotoxin challenge (Nesic et al. 2021).
Environmental factors include for example temperature, humidity, mechanical injury and crop damage by insects (Bryden, W.L. 2012). Temperature is considered to be important in determining which fungi and mycotoxins can grow and infect the crop. Some fungi thrive better in low temperatures, while others grow ideally in higher temperatures. The temperature will therefore determine which mycotoxins will be produced. Aflatoxin risk for example increases with warmer temperatures and reduces when temperatures are lower, all because A. flavus thrives well under heat (Perrone et al. 2020). In general, it can be stated that fungi in the field need more moisture for growth than during storage. The suggested moisture levels needed for growth are about 22-25% of wet weight. This moisture will be used by the fungi for germination of the spores and also for growth (Hesseltine, C.W. 1979).
Mechanical injury of crops causes an increase in susceptibility to fungal invasion. Regardless of the environmental conditions, the growth of fungi is increased when the kernels are injured compared to uninjured kernel (Neme and Mohammed, 2017). The injuries provide an easier entrance for the fungi into the crop (Magan, 2003).
Insects are considered to be a major problem, since they can also damage the crops, making the crops more susceptible to invasion by toxigenic fungi and the associated production of mycotoxins (Chulze, S.N. 2010). They can form kernel wounds, that make it possible for the fungi to enter to the endosperm. Some larvae feed on the kernels and therefore cause damage. Besides, insects also function as vectors that transfer the fungal spores from the surface of a crop, to the inside of the kernel or stalk (Neme and Mohammed, 2017). It is therefore considered very important to reduce (insect caused) damage during the growing season of the crops, but also during drying and storage (Chulze, S.N. 2010).
Harvest
When harvesting the crops, it often occurs that the crops are mechanically injured, allowing for high inoculation of fungal spores (Hesseltine, C.W. 1979). The moment of harvest can affect the amount of mycotoxins in the raw materials. In general, the sooner the harvest, the lower the mycotoxin levels (Jouany, J.P. 2007). For example, for fumonisins in corn, infection can occur in the beginning of ear development, but the mycotoxin production keeps increasing during further development and maturing of the corn ear. This suggests that early harvest can aid in preventing high mycotoxin levels (Warfield and Gilchrist, 1999).
Another important consideration during harvest is the cutting height of the used machinery, since this affects the interaction between the soil (possibly containing new fungi) and the crops. An initial rinsing step should be implemented to eliminate crops that are injured. Also, crops that are clearly infected, for example with foot rot, should be avoided during harvest (Jouany, J.P. 2007).
It was shown that the moisture level of the crops during harvest strongly affect the final production of mycotoxins (Ono et al. 2002). This indicates the importance of the time of harvest; whether the humidity is high (during morning dew or rainfall) or low (in the afternoon) (Jouany, J.P. 2007).
Storage
A distinction can be made between field fungi and storage fungi. In general, the fungi originate from the field, however they can further expand during storage. During storage, fungal production depends on the moisture levels, temperature, composition of the raw materials, and presence of insects. The importance of minimizing access of the ingredients by insects was already discussed, but humidity and temperature are also considered very important in reducing the production of storage mycotoxins. Fungi need humidity levels of more than 65% for growth during storage, and most growth occurs at temperatures between 10 and 40 °C (depending on the type of fungi). By keeping moisture levels and temperatures below these values, mycotoxin production during storage can be minimized (Atanda et al. 2011). In general, crops with moisture levels of over 130 g/kg are prone to the development of fungi and mycotoxins (Brydan, W.L. 2012). Sometimes, it can occur that there are leaks in storage packaging, floods or condensation, which cause the stored goods to become wet, allowing for increased production of mycotoxins. Such events should be avoided at all times (Hesseltine, C.W. 1979).
As already briefly mentioned in the section on the general information on mycotoxins, growth of fungi also depends on the bioavailability of nutrients (Milani, J.M. 2013). In general, it seems that fungi grow best when the substrate contains high levels of carbohydrates (Pitt and Hocking, 2022).
Besides insect damage occurring in the field, insects often attack stored ingredients and can cause an accumulation of moisture, serving as optimal circumstances for the growth of fungi and production of mycotoxins. Keeping insects away from the crops, in all stages of the production process, is an important factor in minimizing mycotoxin levels in the feed (Chulze, S.N. 2010).
The available oxygen and carbon dioxide can also influence the production of fungi during storage. Most fungi need oxygen but there are some efficient oxygen scavengers that can even grow at low levels of oxygen, for example Penicillium expansum. In general the oxygen level, amount of oxygen that is dissolved, is considered to be an important factor for mould growth. High levels of carbon dioxide can reduce fungal growth, however some species are still able to grow under high levels of carbon dioxide, such as Penicillium roqueforti (Pitt and Hocking, 2022).

European guidelines on maximum inclusion levels in feed
Regulations on maximum inclusion levels of mycotoxins in feed differ between countries or regions. In general, the European guidelines (Commission recommendations, 2006/576/EC) are considered to be stricter. Also for the different species, the maximum inclusion level may vary.
For aflatoxins, EU limits range from 5 ppb (compound feed for dairy animals, lambs, goat kids, piglets and young poultry), to 10 ppb (complementary and complete feed) to 20 ppb (all feed materials and compound feed for adult cattle, sheep, goats, pigs and poultry).
Fumonisins have EU limits ranging from 5,000 ppb (complementary and complete feed for pigs, horses, rabbits and pets), to 10,000 ppb for fish, 20,000 ppb (for poultry, calves, goat kids and lambs), to 50,000 for adult ruminants and 60,000 for corn and corn-by-products.
The EU limits for zearalenone vary from 100 ppb (complementary and complete feed for piglets and gilts), 250 ppb (complementary and complete feed for sows and fattening pigs), 500 ppb (complementary and complete feed for calves, dairy cattle, sheep and goats), 2,000 ppb in cereal (products) to 3,000 ppb in corn and corn-by-products.
Ochratoxins have to comply with EU limits of 10 ppb (complementary and complete feed for cats and dogs), 50 ppb (complementary and complete feed for pigs), 100 ppb (complementary and complete feed for poultry) to 250 ppb (cereal (products)).
EU legislation for T-2 and HT-2 states limits of 50 ppb (compound feed for cats), 250 ppb (compound feed), 500 ppb (cereal products for (compound) feed) to 2,000 ppb (oat milling products for (compound) feed).
Finally, for DON, EU limits range from 900 ppb (complementary and complete feed for pigs), 2,000 ppb (complementary and complete feed for dogs, calves, lambs and goat kids), 5,000 ppb (complementary and complete feed), 8,000 (cereal (products)), to 12,000 (corn-by-products).

In-feed solutions
A wide variety of products, intended to reduce the negative effects of mycotoxins, are globally available. A common mode of action for such products is the binding of mycotoxins, also called adsorption (Kolosova and Stroka, 2011). Clay minerals are considered to be natural adsorbents of mycotoxins, and are generally available at quite low prices. Montmorillonite, bentonite and zeolite are examples of clays that have the capacity to bind aflatoxins in the gastrointestinal tract, and therefore reduce the absorption of aflatoxins. Such clays are generally non-nutritive and non-toxic for the animals, but allow for a protective effect against certain mycotoxins (Oguz and Kurtoglu, 2000). Besides the inorganic adsorbents, such as zeolites, bentonites and other clays, organic binders can be used, for example yeast cell wall constituents (Kolosova and Stroka, 2011). In general, it can be stated that mycotoxin binders can vary in efficiency, depending on the mycotoxins
in the feed, as well as on the binder itself. Polarity, size, solubility, shape and charge are important characteristics of the mycotoxins, that determine the efficacy to which they can be adsorbed by the binder product. Also the external pH, of the environment to which the mycotoxin binder is added, is important in the adsorption process (Dakovic et al. 2005). Clays can be defined as naturally occurring minerals with a particle size smaller than 2µm (Subramaniam and Kim, 2015). Most types of clays are phyllosilicates, meaning that they consist out of layers. Such phyllosilicates can be subdivided into two groups, depending on the number, type and charge of the layers. The kaolin group (for example nacrite, kaolinite and dickite) have a 1:1 structure, with a tetrahedral Si sheet that is linked to an octahedral Al sheet via a covalent bond (Subramaniam and Kim, 2015). Another type of structure is the 2:1 group, consisting out of one octahedral sheet (generally Mg, Al or the combination of Mg and Al) fitting in the middle of two tetrahedral Si sheets. This type of structure is called the smectite group (for example montmorillonite, saponite or hectorite) (Subramaniam and Kim, 2015). Next to phyllosilicates, another type of clay can occur with a three-dimensional structure. This group is called the zeolites, and the three dimensional structure (of SiO44− , and AlO45-) is linked via shared oxygen atoms (Subramaniam and Kim, 2015). Via the three-dimensional pores, exchange of cations and water can occur. These clays are negatively charged, making them very effective cation-exchangers for positively charged toxins. Due to the small pore size of zeolites, they are considered to be very selective in adsorption, with high affinity for toxins and other contaminants (Subramaniam and Kim, 2015).
Orffa developed Excential Toxin A as a single-spectrum solution, containing one ingredient and aiming at binding the mycotoxins in the feed. The product consists of one specific zeolite, tectosilicate (clinoptilolite), which is mined in Europe. As a zeolite, it has the typical 3-dimensional frame work with small pores, as mentioned above. The focus of Excential Toxin A is to bind the mycotoxins in the gastrointestinal tract of the animal, in order to prevent the mycotoxin from being absorbed on an intestinal level and entering the bloodstream.
The product has been shown to have complete binding of aflatoxins (>90%), both at pH 3 (simulating the stomach environment) as well as pH 7 (simulating the intestinal environment). Also for the enniatins, part of the emerging mycotoxins, binding was shown to be over 90% in the full pH range of the gastrointestinal tract. For fumonisins, the product shows complete binding at pH 3, but strongly reduced binding at pH 7.
When, besides aflatoxins, fumonisins or enniatins, a) other types of mycotoxins are present in the feed, b) when contamination levels are high or c) there’s co-contamination, it’s advised to switch from Excential Toxin A to Excential Toxin Plus. Excential Toxin Plus is Orffa’s broad-spectrum mycotoxin adsorbent, aimed at the full spectrum of mycotoxins.
This product consists out of five ingredients, and focusses on five functions to reduce the negative effects of mycotoxins. First of all, the product includes organic acids aimed at preventing the growth of mould and mycotoxins in stored feed. Literature shows that inclusion of calcium propionate can reduce the growth of Aspergillus flavus, and reduce the production of AFB1 by Aspergillus flavus (Alam et al. 2009).
As second function, the product aims at the adsorption of mycotoxins in the gastrointestinal tract of animals, via the inclusion of two aluminosilicates and yeast in the product. The combination of these three ingredients allows for a synergistic effect, with high binding efficacy for different types of mycotoxins.
The third function is considered to be strengthening the intestinal barrier via the inclusion of betaine in Excential Toxin Plus. Many mycotoxins, such as DON, reduce villi length intestinal barrier function (Pinton et al. 2012), causing the animal to be more susceptible to pathogens. Betaine can accumulate in intestinal cells, and is known for having a protecting effect on the intestinal cells during a challenge (Kettunen et al. 2001). In research published by Kettunen et al. (2001), it was shown that betaine can reduce the harmful effect of a challenge on intestinal villi, allowing for longer villi during challenge compared to the challenged group without betaine.
A recent trial by Orffa showed that, when challenging CACO-2 cells with DON, the trans-epithelial electrical resistance (TEER) decreases. The inclusion of betaine during this challenge with DON, allows for a protective effect, shown by the higher TEER compared to the challenged group without betaine (Figure 1).

The liver is an organ that is often negatively affected by mycotoxins in the diet (Domijan and Peraica, 2010). Besides accumulation in intestinal cells, betaine is also known to accumulate in liver cells (Kettunen et al. 2001). Wen et al. (2021) showed that betaine allows for improved liver health in broilers fed mycotoxin (zearalenone) contaminated feed. The fourth function of Excential Toxin Plus can therefore be stated to be the hepatoprotection by betaine.
Finally, as described in the first section of this paper, mycotoxins often reduce immune functioning (Sobrova et al. 2010, Tao et al. 2018). Yeast has been extensively described in literature for its immune stimulating effects (El-Boshy et al. 2010). Therefore, the fifth function of Excential Toxin Plus is to strengthen the overall immune function by the inclusion of yeast. This yeast therefore has a dual function in the product; adsorbing mycotoxins and immune support.
Some mycotoxins, such as the trichothecenes, are known to be difficult to bind as shown in the in vitro trial studying binding efficacy (Table 1). For such mycotoxins, it is important to include other strategies that contribute in reducing negative effects of these mycotoxins. Excential Toxin Plus is a broad-spectrum solution, with functions not only aimed at adsorption, but also aimed at prevention, intestinal support, hepatoprotection and strengthening of the immune system. It can therefore provide protection against the full range of mycotoxins.

Excential Toxin A Trial data
In collaboration with the research facilities of Arte Casearia in Modena, Italy, which is the official Institution of the Parma cheese producers, a trial was performed to investigate the effects of Excential Toxin A in dairy cows. In total, 300 dairy cows were included in this study, following aflatoxin levels in milk during five subsequent phases 1) Natural level of AFB1 in feed (day 0-1), 2) Natural contamination of feed with AFB1 (days 1-15), 3) Natural contamination of feed with AFB1 + 100 g Excential Toxin A per cow per day (days 15-32), 4) Natural contamination of feed with AFB1 + 200 g Excential Toxin A per cow per day (days 32-45), 5) Natural contamination of feed with AFB1 (days 45-57). The trial results (Figure 2) show that supplementation with 100 g/cow/day of Excential Toxin A, when AFB1 level in the feed is approximately 2.49 ppb, allows for a reduction in the AFM1 levels in the milk from about 85 ppb to 12.5 ppb. Increasing the dosage from 100 g/cow/day to 200 g/cow/day was not shown to further reduce AFM1 levels in the milk.

Publications Excential Toxin Plus
Trial data binding efficacy
In collaboration with the Centre of Excellence in Mycotoxicology and Public Health at the University of Ghent (Belgium), Orffa designed an in vitro model to analyse the binding capacity of different commercial mycotoxin adsorbents, using liquid chromatography with tandem mass spectrometry (LC-MS/MS). The assay mimics the conditions in the gastrointestinal tract by testing the compounds both at pH 3 (which represents the pH level in the stomach) as well as pH 3-7 (resembling the pH in the intestine). This trial compared the binding capacity of the 8 premium mycotoxin binders that are globally available, to the binding efficacy of Excential Toxin Plus for different mycotoxins: trichothecenes (DON, HT-2, T-2), zearalenone (ZEN), aflatoxins (AFB1, AFB2, AFG1, AFG2), ochratoxins (OTA), and fumonisins (FUM B1, FUM B2) (published at the World Mycotoxin Forum Amsterdam, 2018).
The binding efficacy for the trichothecenes (DON, HT-2, T-2) is considered to be low, which is also shown in the results of this trial (Table 1). It is shown that the adsorption capacity for the trichothecenes is low for all tested products. Aflatoxins on the other hand, are shown to be bound to a high extent for all of the tested products, both at pH 3 as well as pH 3-7. Some of the tested products are shown to bind ochratoxins, but in general binding capacity at pH 7 is quite low for this type of mycotoxin. For fumonisins, quite similar results were found, also showing that some products have high binding capacity at pH 3, but low binding at pH 7.
When considering Excential Toxin Plus, the product shows equal or better binding efficacy compared to 8 commercially available premium mycotoxin binders.

Trial data for emerging mycotoxins
An in vitro trial (published at ASMMytox conference, 2022) studied the binding efficacy of Excential Toxin Plus towards alternariol and enniatin B. The trial was performed at the University of Ghent, using a similar set-up as for the previous trial. It was shown (Table 2) that there is complete binding (>91%) of Excential Toxin Plus towards alternariol at both pH 3 and pH 3-7. For enniatin B, there was partial binding (51-90%) at pH 3 and complete binding (>91%) at pH 3-7.
Overall, it can be concluded that Excential Toxin Plus can completely bind the emerging toxins alternariol and enniatin B in the pH range of the gastrointestinal tract (pH 3-7). This indicates that it can protect animals against negative effects of these mycotoxins.


Trial data in poultry – Laying Hens
In collaboration with the CERSA and the University of Lomé in Togo, a trial in laying hens was performed to study the effects of Excential Toxin Plus in an aflatoxin-contaminated layer diet (published at World Poultry Congress, Paris 2022). The trial included 840 hens, in cages (3 per cage), in open houses, that were randomly assigned to 24 pens. There were two treatments; T1: control diet, T2: control diet + 1.5 kg of Excential Toxin Plus / ton of feed.
It was shown (Table 3) that Excential Toxin Plus significantly improved egg production, especially in old hens (+8.7%, p<0.05); +2.4% for young hens), and significantly reduced FCR for both old (-9.2%, p<0.05) and young (-4.6%, p<0.05) hens. In old hens, Excential Toxin Plus was shown to improve egg weight by +4.0 g, due to a significant improvement in albumen and shell weight (p<0.05).


Trial data in poultry – Broilers
Another poultry trial in collaboration with the Ecole Inter-Etats des Sciences et Médecine vétérinaires (EISMV) in Senegal, was performed in broilers (published at World Poultry Congress, Paris 2022). Six hundred Cobb 500 broiler chickens, non-sexed were included in the trial. The first 10 days, all birds received the same control diet. At day 11, the birds were randomly divided over 3 treatments; T1: control diet, T2: control diet + 1 kg Excential Toxin Plus / ton of feed, T3: control diet + 5 kg Excential Toxin Plus / ton of feed. The raw cake used to formulate these diets was analyzed on aflatoxin levels, which were shown to be 160.4 µg/kg.
The use of 1 kg/ton Excential Toxin Plus was shown (Figure 3) to result in a 2.4% increase in final body weight (p<0.01) and a 5.6% reduction in FCR. The use of 5 kg/ton of Excential Toxin Plus did not affect final body weight but was shown to reduce FCR by 4.0%. It was hypothesized that under commercial circumstances, where the levels of aflatoxins in the feed are higher and different mycotoxins co-occur, the effects of Excential Toxin Plus will be more apparent.
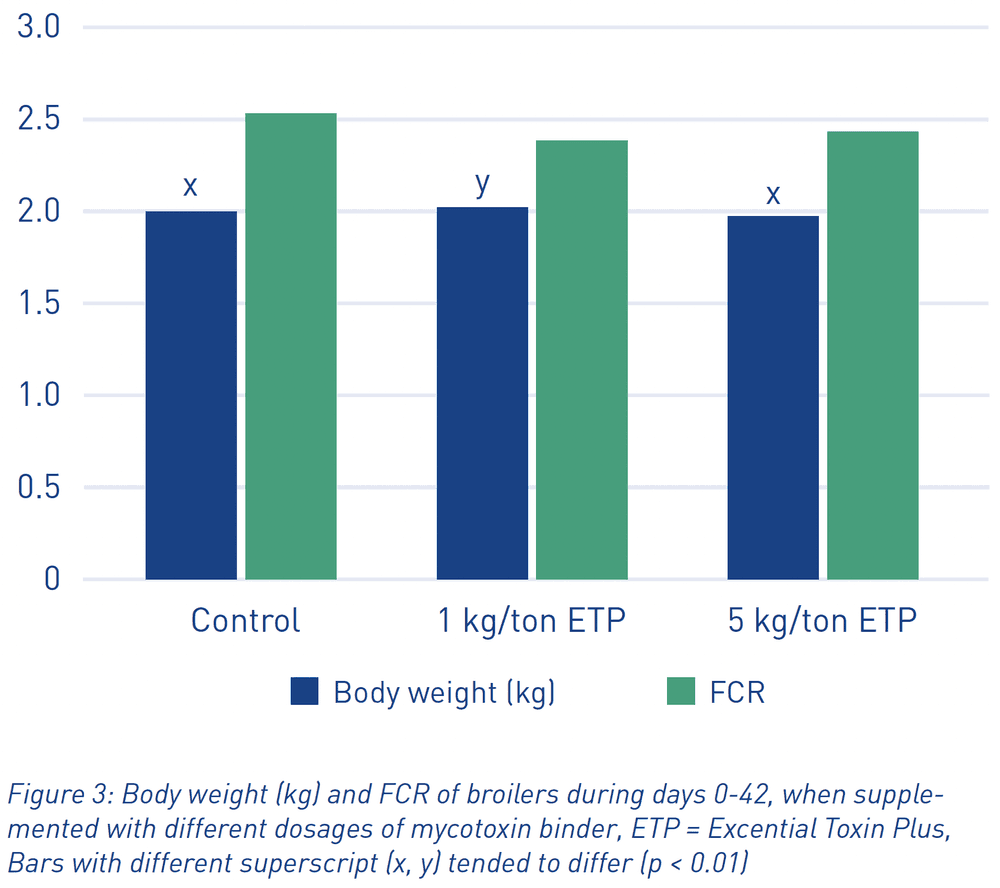
Trial data in swine
A recent trial (published at World Mycotoxin Forum Parma, 2022), performed on a commercial farm in the Philippines, investigated the effects of three different broad-spectrum mycotoxin binders on reducing the effects of zearalenone on growth performance and incidence of Vulva Hypertrophy (VH) in gilts. The study consisted out of two trials, T1 performed in nursery gilts and T2 in growing gilts. Both trials started when the gilts showed signs of VH. Gilts from the same batch were allocated into three groups, fed three different types of multicomponent mycotoxin adsorbents A, B or Excential Toxin Plus. For both trials, weight and feed intake were recorded and feed conversion ratio (FCR) was calculated.
Vulva hypertrophy was noted per animal, initially and at the end of the trial period. Both trials lasted until the gilts were 180 days old and were selected, either as replacement gilts or sold as finishers, with selection criteria being the occurrence of estrous during these first 180 days.
In both trials, considering the overall period, gilts from the Excential Toxin Plus group had higher final body weight and daily gain, and lower FCR compared to groups A and B (Figure 4). The Excential Toxin Plus group also resulted in the highest reduction of VH cases (-54%) in T1. In T2, groups B and Excential Toxin Plus reduced VH cases in more than 30%, with group A increasing appearance of VH.

Overall, the inclusion of Excential Toxin Plus to the diets of replacement gilts resulted in improved growth performance and reduced signs of vulva hypertrophy. These results indicate that this mycotoxin adsorbent reduces the negative effects of zearalenone on reproductive and growth performance to a larger extend, compared to the two others adsorbents.
Conclusions
Mycotoxins are already present as a major challenge for animal production worldwide, accounting for substantial economic losses. Due to the changing climate and intensive agricultural practices, the mycotoxin challenge is expected to increase in the coming years. Not only the most commonly known mycotoxins, such as aflatoxins, fumonisins, zearalenone, ochratoxins, DON, T-2 and HT-2 present a challenge, but also the emerging mycotoxins. This highlights the need for more research into toxicity mechanisms, also into means for mycotoxin prevention and reduction. The market for mycotoxin solutions already includes different commercial available products. Orffa developed Excential Toxin A, a single spectrum solution focussed mainly on the binding of aflatoxins and fumonisins. A second product, Excential Toxin Plus, is a broad spectrum solution with high in vitro binding efficacy to different type of mycotoxins, and on top, prevention, intestinal support, hepatoprotection and strengthening of the immune system. Both products have been demonstrated to reduce the negative effects of mycotoxins in vivo.